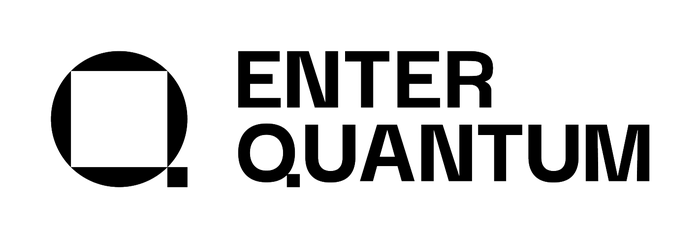
Connects decision-makers and solutions creators to what's next in quantum computing
Overcoming the Errors Holding Quantum Computing Back
The quest to reduce quantum computing errors is a fundamental step toward unlocking the transformative potential of this technology
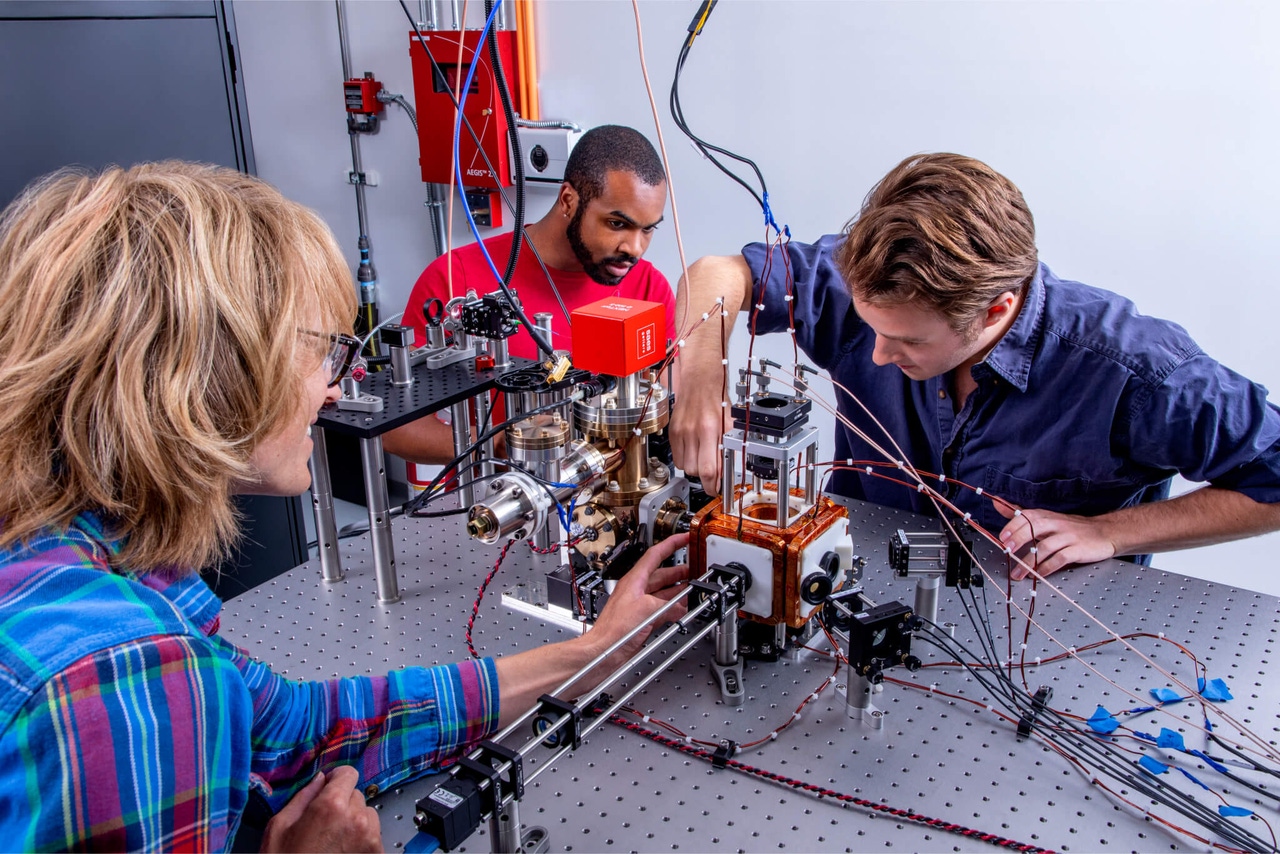
Quantum computers have the potential to tackle calculations that are currently impossible for classical computers, promising transformative impacts across various industries. However, one formidable barrier stands in the way of realizing this potential—the high error rate in quantum computations.
Understanding Quantum Errors
Qubits, the basic units of quantum computing, are inherently fragile and highly susceptible to external disturbances. Unlike classical bits, which are either 0 or 1, qubits can exist in a superposition of states, representing both 0 and 1 simultaneously. This quantum behavior allows for powerful parallel computations but also makes qubits extremely sensitive to their environment. Even the slightest interaction with external factors—such as thermal fluctuations, electromagnetic interference, or stray atoms—can cause a qubit to lose its delicate quantum state, leading to computational errors that undermine the results.
These errors manifest as unintended changes in a qubit’s state and can significantly limit the size, complexity and reliability of quantum algorithms. To achieve practical quantum computing, we need effective strategies to mitigate these errors and maintain the integrity of quantum information over time.
The Challenge of Quantum Error Correction
Addressing quantum errors requires sophisticated error correction techniques. Unlike classical error correction, which typically involves simple redundancy (e.g., repeating data bits), quantum error correction must cope with the quantum nature of information, where qubits can exist in entangled states and cannot be copied directly due to the no-cloning theorem.
Quantum error correction (QEC) involves encoding a logical qubit—a robust unit of quantum information—into a set of physical qubits. By spreading the quantum information across multiple qubits, QEC can detect and correct errors without measuring the qubits directly, which would collapse their quantum states. This process, while complex, allows for more prolonged and accurate quantum computations.
There are various methods for constructing logical qubits, each with its own advantages and challenges. A common technique is the “stabilizer code,” such as the surface code, which organizes qubits into a lattice structure and uses a series of measurements to detect errors. The surface code is particularly promising because it requires only local interactions between neighboring qubits, making it more feasible for current quantum hardware.
Innovations in Quantum Error Correction Techniques
The development of error correction methods is closely tied to the hardware used to implement qubits. Several types of quantum computing architectures are being explored, including superconducting circuits, trapped ions and neutral atoms.
One promising approach is the use of neutral atoms as qubits. In this method, each qubit is a single atom, controlled by precisely tuned laser beams. Neutral-atom systems can operate at temperatures much closer to room temperature compared with superconducting qubits, which require near-absolute-zero temperatures. This feature can simplify integration into existing data centers and reduce some forms of environmental interference. Additionally, neutral-atom platforms offer exceptional scalability, with the expected ability to control tens of thousands of atoms on a single machine. This scalability is crucial for advancing quantum computing, as it allows for the creation of large, highly connected quantum systems capable of tackling more complex problems and running more sophisticated algorithms.
A significant advantage of neutral-atom systems is the ability to move qubits around, a process known as “qubit shuttling.” This capability allows for dynamic reconfiguration of qubits, optimizing their positions for specific computations and minimizing errors from unwanted interactions. Qubit shuttling facilitates the implementation of transversal gates, which are essential for error correction because they can operate on multiple qubits simultaneously without spreading errors. Additionally, neutral-atom systems enable parallel operations, where several qubit pairs can perform gates concurrently, significantly speeding up computations. These features lead to shorter, more efficient algorithms with fewer operations, thereby reducing the accumulated error rates and improving overall quantum computational fidelity.
Quantum Low-Density Parity-Check Codes
Quantum Low-Density Parity-Check (QLDPC) codes are a promising type of error-correcting code that has gained attention for their potential to provide robust error correction with lower overhead compared to traditional methods like surface code. QLDPC codes use a sparse parity-check matrix to define the relationships between qubits, allowing for efficient error detection and correction with fewer qubits needed for redundancy. This can significantly reduce the resource demands on quantum hardware, making it easier to scale up quantum systems. The sparsity of the parity-check matrix in QLDPC codes enables faster and more efficient decoding algorithms, which can be particularly advantageous for quantum computers with limited connectivity between qubits. As research into QLDPC codes progresses, they could become a key tool in achieving scalable and fault-tolerant quantum computing. QLDPC codes are very well-suited for neutral-atom computers
Path to Scalable Quantum Computing
Overcoming qubit errors is crucial for making quantum computers practically useful. Quantum error correction is at the heart of this effort and recent advancements highlight the progress being made. For instance, a recent study led by Harvard, in which QuEra played a pivotal role, demonstrated an increase in the number of logical qubits from two or three to 48. This achievement is significant because it pushes the boundaries of what classical computers can simulate, bringing us closer to quantum supremacy—a point where quantum computers outperform the most powerful classical counterparts on specific tasks.
While the road to scalable, fault-tolerant quantum computers is still long, these advances underscore the potential for quantum technology to solve problems once thought intractable. As research continues to improve both qubit hardware and error correction techniques, we move steadily towards a future where quantum computing can fulfill its promise across various industries, from drug discovery to cryptography and beyond.
Paving the Way for Quantum Breakthroughs
The quest to reduce quantum computing errors is more than a technical challenge; it’s a fundamental step toward unlocking the transformative potential of this technology. By leveraging innovative error correction strategies and advances in qubit control, researchers and companies like QuEra are paving the way for a new era of computing. As the field progresses, the promise of quantum computing—to solve the world’s most complex problems—becomes increasingly within reach.
About the Author
You May Also Like